Design strategy
The DAGQD@Cu SAN was synthesized using a DAGQD template. Subsequently, KGN, a small molecule that induces mesenchymal stem cell differentiation into chondrocytes, was grafted onto the surface of the DAGQD@Cu SAN (DAGQD@Cu@KGN SAN) using the amide reaction (Fig. 1A). In this process, catechols of DA act as anchoring sites that enhance the interaction between DAGQD and Cu2+, allowing Cu single atoms to uniformly disperse on the surface of DAGQD. Moreover, catechols in DA can improve the ROS-scavenging ability of SAN.
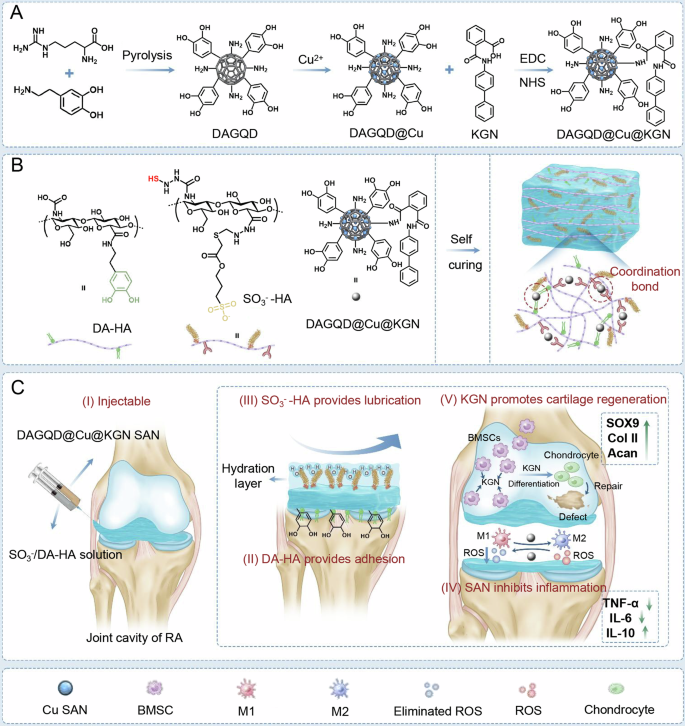
A Synthesis of DAGQD@Cu SAN and DAGQD@Cu@KGN SAN. B Synthesis of DAGQD@Cu@KGN-SO3−/DA-HA hydrogel. C DAGQD@Cu@KGN-SO3−/DA-HA hydrogel for RA therapy. (I) Hydrogel self-cures in situ within the joint cavity; (II, III) DAGQD@Cu@KGN-SO3−/DA-HA hydrogel displays lubrication and adhesion properties; (IV) Regulation of inflammatory microenvironment facilitated by SAN with remarkable ROS-scavenging capabilities; (V) KGN released from the hydrogel promotes the recruitment of BMSCs and induces their differentiation into chondrocytes, facilitating the repair of cartilage damaged by RA.
The sol to gel transition of the injectable hydrogel was triggered by DAGQD@Cu SAN, acting as the crosslinking points via metal coordination between -SH in SO3−-HA and catechol in DA-HA networks, along with Cu2+ in DAGQD@Cu SAN (Fig. 1B). This unique property allowed the hydrogel to be injected into the joint cavity and undergo self-curing in situ (Fig. 1CI). Simultaneously, bioadhesion and lubrication were achieved through the enhanced complementary hydrogel network enabled by DAGQD@Cu SAN. This network integrated the adhesive properties of the DA-HA network for cartilage surface attachment with the wear reduction and lubrication enhancement capabilities of the SO3−-HA network (Fig. 1CII and III). Consequently, this mechanism helped mitigate cartilage wear caused by elevated friction coefficients in RA models.
The bioadhesiveness of the hydrogel originates from DAGQD@Cu SAN and DA-HA, drawing inspiration from mussel adhesion mechanisms. Meanwhile, the lubricity of the SAN-SO3−/DA-HA hydrogel stems from SO3−-HA, inspired by the composition of synovial fluid. Synovial fluid within joints comprises HA, lubricating proteins, lipids, globulin, albumin, and glycosaminoglycans. Among these components, HA, lubricating proteins, and lipids amalgamate into lubricating complexes that form a stable hydration layer, termed the lubrication layer, on cartilage surface, effectively preventing cartilage wear. Furthermore, the GAGQD@Cu SAN embedded in the hydrogel mitigated the inflammatory microenvironment within the RA-affected joint cavity due to its outstanding ROS-scavenging activity (Fig. 1CIV). Moreover, the release of KGN from the hydrogel facilitated the recruitment of endogenous host bone marrow mesenchymal stromal cells (BMSCs), promoting homing and inducing BMSCs differentiation into chondrocytes for the repair of cartilage damaged by RA (Fig. 1CV).
Characterization of SAN
The DAGQD appeared as monodisperse black dots measuring ~5 nm in size. Analysis revealed a lattice spacing of 0.21 nm, corresponding to the (001) crystal plane of graphite carbon (Supplementary Fig. 1). Upon integration with Cu, the DAGQD@Cu SAN exhibited a monodisperse spherical shape with a size ranging ~5–10 nm (Fig. 2A). The slightly increased size could be attributed to Cu chelation with catechol on the DAGQD. HRTEM imaging indicated a lattice spacing of ~0.25 nm, aligning with the (100) crystal plane of graphite carbon (Supplementary Fig. 2A). This suggested that the introduction of a small amount of Cu minimally affected the structure of the DAGQD. Comparatively, the size of DAGQD@Cu@KGN SAN remained largely unchanged from DAGQD@Cu SAN (Fig. 2B), maintaining a lattice spacing of ~0.25 nm (Supplementary Fig. 2B). KGN grafting did not alter the size or structure of the DAGQD@Cu SAN. Notably, HAADF-STEM imaging depicted atomically dispersed Cu single atoms within the DAGQD@Cu SAN (Fig. 2C). Moreover, XRD analysis did not reveal significant diffraction peaks of crystalline Cu NPs, further corroborating the presence of single Cu atoms (Supplementary Fig. 3).
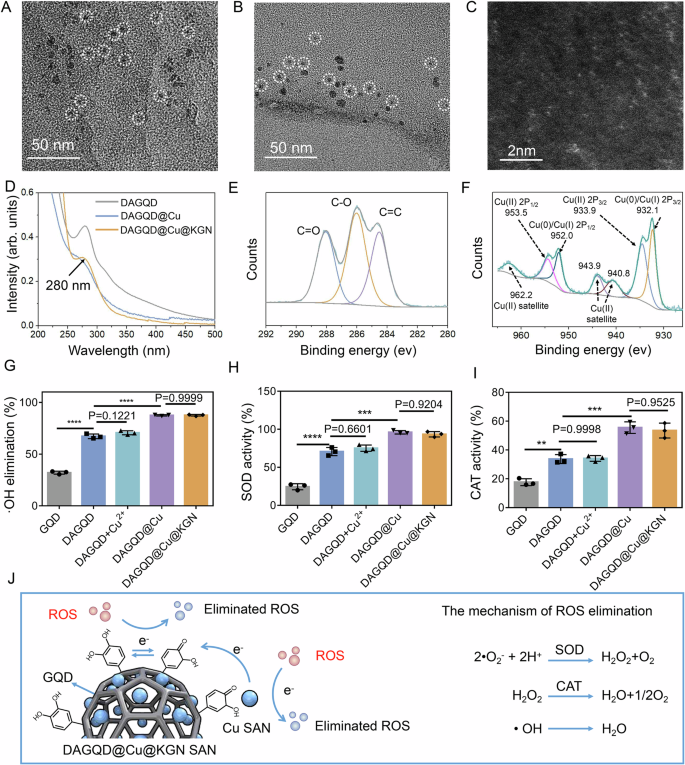
TEM images of (A) DAGQD@Cu SAN and (B) DAGQD@Cu@KGN SAN. C High-Angle Annular Dark-Field Scanning Transmission Electron Microscopy (HAADF-STEM) image of DAGQD@Cu SAN. D The UV-Vis spectrum of DAGQD, DAGQD@Cu SAN, and DAGQD@Cu@KGN SAN. E The C1s spectrum and (F) Cu2p spectrum of DAGQD@Cu SAN. G Hydroxyl radical scavenging ability, (H) SOD activity, and (I) CAT activity of GQD, DAGQD, DAGQD+Cu2+, DAGQD@Cu SAN, and DAGQD@Cu@KGN SAN (n = 3 samples). J The mechanism of ROS elimination of DAGQD@Cu SAN. (Data are presented as the mean ± SD. Statistical analysis was performed using one-way ANOVA followed by Tukey’s post-hoc test. *, **, and **** indicate significance at p < 0. 05, p < 0.01, and p < 0.0001, respectively).
The UV–Vis absorption spectrum of DAGQD displayed a shoulder peak at 280 nm and a notably robust characteristic absorption peak at 315 nm. This peak was attributed to the π-π* electronic transition of conjugated C = C units within the carbon core and n-π* transition of the C = O bond, respectively26. The absorbance of DAGQD@Cu SAN at 280 nm and 315 nm proved lower than that of DAGQD, illustrating efficient chelating interactions between Cu(II) and surface functional groups such as catechol and carboxylic groups on the DAGQD27. Moreover, the absorbance of DAGQD@Cu@KGN SAN at 280 nm surpassed that of DAGQD@Cu SAN, attributed to the distinctive absorption peak of KGN (Fig. 2D).
X-ray photoelectron spectroscopy (XPS) results showed characteristic peaks at 285 and 288 eV, corresponding to the C-O and C = O bonds representing the catechol and quinone groups in the DAGQD@Cu SAN, respectively (Fig. 2E, Supplementary Fig. 4, 5). The Cu2p spectrum of DAGQD@Cu SAN exhibited multiple peaks, with the peaks at 932.2 and 952.0 eV were attributed to Cu0/Cu(I)2p. Furthermore, two peaks at 933.9 and 953.5, together with satellite peaks around 940.8, 943.9, and 962.2 eV were indicative of Cu(II)2p (Fig. 2F). Notably, DAGQD@Cu@KGN SAN displayed the same copper valence state, indicating that the structure of DAGQD@Cu remained unchanged after the introduction of KGN (Supplementary Fig. 6). The grafting degree of KGN in DAGQD@Cu SAN was 58.6%, and the load rate of KGN in DAGQD@Cu SAN was 1.1%. These findings confirmed the successful introduction of KGN into the DAGQD@Cu SAN.
ROS-scavenging activities of SAN
The DAGQD@Cu@KGN SAN exhibited high enzyme-like activity in scavenging ROS (Fig. 2G–I). DAGQD displayed higher SOD and CAT activities along with enhanced clearance rates for •O2− and •OH than that of GQD, owing to the present of catechol groups in DAGQD. To establish the enhanced ROS-scavenging potential of DAGQD@Cu SAN with multivalent copper over DAGQD with single-valent copper, we combined DAGQD and CuCl2 solutions, recorded as DAGQD+Cu2+. Results showed that DAGQD@Cu SAN displayed superior ROS-scavenging and CAT activities compared with DAGQD and DAGQD+Cu2+. The exceptional catalytic activity can be ascribed to the variable valence of copper single atoms and the abundant catechol structure. Furthermore, no significant difference was observed in ROS scavenging and CAT activities between DAGQD@Cu SAN and DAGQD@Cu@KGN SAN, suggesting that the introduction of KGN did not disrupt the structure of DAGQD@Cu SAN. In addition, DAGQD@Cu SAN has excellent stability (Supplementary Fig. 7).
The high catalytic activity of the DAGQD@Cu SAN was attributed to a dual catalytic mechanism. First, single Cu atoms exhibit SOD- and CAT-like activities, catalyzing •O2−, •OH, and H2O2 to produce nontoxic water and O2. Second, electrons were transferred from the single Cu atom to the quinone groups of DA, forming a catechol-quinone redox pair, endowing the system with sufficient catechol groups for ROS elimination (Fig. 2J).
SAN-induced hydrogel gelation
DAGQD@Cu@KGN SAN rapidly initiated the gelation of the SO3−/DA-HA hydrogel due to the metal coordination bond between -SH and Cu (Figs. 3A, Supplementary Fig. 8, 9). Mixing DAGQD@Cu@KGN SAN and SO3−/DA-HA precursor solutions and continuously injecting them into a culture dish using a 1 mL syringe and 0.5 mm needle, filamentous strips can be observed suspending in the air and the formation of the two letters “HY” in the culture dish (Fig. 3B). Rheological measurements indicated that the gelation time of the hydrogel could be adjusted by changing the SAN concentration (Supplementary Fig. 10). The gelation time decreased with increasing SAN content, reaching 21 s at SAN concentration of 1 mg mL−1 (Fig. 3C). Moreover, the hydrogel was gelatinized in situ within the defective cartilage area (Fig. 3D).
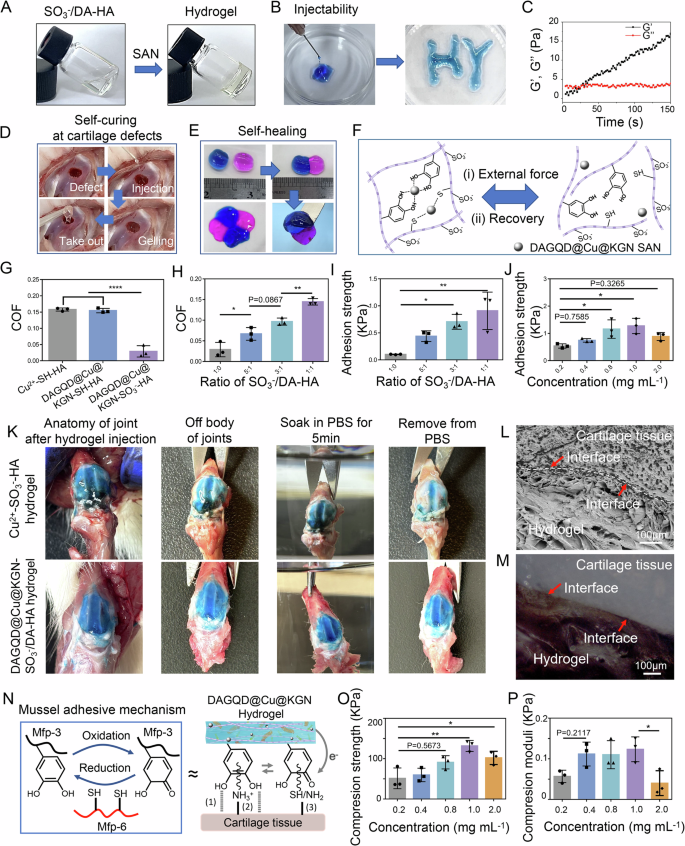
A Photographs depicting the self-curing process of SO3−/DA-HA hydrogel precursor initiated by DAGQD@Cu@KGN SAN. B The injectable property of DAGQD@Cu@KGN-SO3−/DA-HA hydrogel. C Rheological properties of DAGQD@Cu@KGN-SO3−/DA-HA hydrogel. D Images illustrating the self-curing process of DAGQD@Cu@KGN-SO3−/DA-HA hydrogel in the cartilage defect of a rabbit knee joint. E Hydrogel self-healing ability. F Schematic depiction of the self-healing mechanism. G Coefficient (COF) comparison between Cu2+-SH-HA hydrogel, SAN-SH-HA hydrogel, and SAN-SO3−-HA hydrogel (n = 3 samples). H COF variation of SAN-SO3−/DA-HA hydrogel with different ratios of SO3−-HA and DA-HA (n = 3 samples). I Adhesion strength of SAN-SO3−/DA-HA hydrogel with different ratios of SO3−-HA and DA-HA on cartilage samples (n = 3 samples). J Adhesion strength of the hydrogel with different concentrations of SAN on cartilage samples (n = 3 samples). K Anatomic picture of hydrogel adhesion in joint. L The FESEM and (M) metallographic microscopy image of hydrogel-cartilage cross-section (n = 3 independent experiments). N Illustration of the bioadhesion mechanism of DAGQD@Cu@KGN SAN hydrogel on cartilage tissue, involving (1) hydrogen bonds, (2) cation-π interaction, and (3) covalent linking. O Compressive strength and (P) compression modulus analysis of the hydrogel with varying concentrations of DAGQD@Cu@KGN SAN (n = 3 samples). (Data are presented as the mean ± SD. Statistical analysis was performed using one-way ANOVA followed by Tukey’s post-hoc test, with a value of *P < 0.05, **P < 0.01, and ****P < 0.001).
The DAGQD@Cu@KGN-SO3−/DA-HA hydrogel also exhibited self-healing effects (Fig. 3E). The hydrogels were dyed blue and red, then combined. The two hydrogels fused into one after incubation at 37 °C for 2 min. The red hydrogel did not fall down when lift the blue one with tweezer. Placing the four hydrogels in order and incubating them at 37 °C for 2 min resulted in a round, well-fused structure. These results indicate the favorable self-healing properties of the hydrogel. The self-healing properties of the DAGQD@Cu@KGN-SO3−/DA-HA hydrogel were analyzed using rheological measurements. The G’ of the hydrogel was significantly higher than the G” under low strain, indicating hydrogel formation. With increases strain, the hydrogel transitioned to a fluid state, with a critical strain value of ~550% (Supplementary Fig. 11A). Dynamic step strain measurement revealed that the hydrogel behaved colloidal (G’ > G”) at a low shear rate 10%. However, under a strain of 600%, the hydrogel network was swiftly disrupted (G’ < G”). Upon cessation of the high shear rate, the G’ and G” of the hydrogel recovered to 100% within seconds (Supplementary Fig. 11B). The robust self-healing ability of the DAGQD@Cu@KGN-SO3−/DA-HA hydrogel stemmed from the dynamic and reversible bonds of-SH/DA and Cu2+ in SAN (Fig. 3F). Under shear force, the metal coordination bond broke, rendering the hydrogel flowable. Upon removal of the shear force, the metal coordination bond restored, reverting the hydrogel to a solid state. The self-healing performance of hydrogels proves essential for frequently manipulated joints.
In vitro lubrication and adhesion of the hydrogels
The hydrogel exhibited commendable lubricity due to its SO3−-HA network. Friction coefficients (COF, frictional load was at 1 N, the setting amplitude at 5 mm, sliding frequency at 1 Hz) of the Cu2+-SH-HA, DAGQD@Cu@KGN-SH-HA, and DAGQD@Cu@KGN-SO3−-HA hydrogels were measured (Fig. 3G). Results showed COF values of 0.157 for Cu2+-SH-HA hydrogels, 0.154 for DAGQD@Cu@KGN-SH-HA, and 0.028 for DAGQD@Cu@KGN-SO3−-HA hydrogels. The COF of DAGQD@Cu@KGN-SO3−-HA was significantly lower than that of the other non-sulfate modified hydrogels. Furthermore, the SO3−-HA solution also demonstrated a lower COF in comparison to PBS and SH-HA solution (Supplementary Fig. 12). This suggests that introducing -SO3− significantly enhances hydrogel lubricity.
The hydrogel displayed both lubrication and bioadhesion after the incorporation of DA-HA. Investigating hydrogel lubrication and bioadhesion involved varying the ratio of SO3−-HA and DA-HA. Results revealed an inverse correlation with an increase in the DA-HA ratio. The hydrogel lubrication performance decreased as the proportion of DA-HA increased (Fig. 3H). Conversely, the adhesion of the DAGQD@Cu@KGN-SO3−/DA-HA hydrogel significantly improved with an increased ratio of DA-HA to SO3−-HA, and the adhesion strength was optimal when SO3−-HA: DA-HA = 1:1 (Fig. 3I, Supplementary Fig. 13). However, at a 1:1 ratio of SO3−-HA: DA-HA, the precursor solution displayed extremely poor flowability, rendering it to be injected through the needle (Supplementary Fig. 14). Therefore, the optimal ratio for preparing bioadhesive and lubricating hydrogels appears to be SO3−-HA: DA-HA = 3:1. The adhesion strength (Figs. 3J, Supplementary Fig. 15) of the hydrogel increased with raised SAN content from 0.2 to 1.0 mg mL−1. However, hydrogel adhesion strength decreased when the SAN concentration was further increased to 2.0 mg mL−1. Thus, hydrogels with optimal adhesion properties were obtained at a SAN concentration of 1.0 mg mL−1.
The hydrogel can well adhere to the surface of the rat’s joints (Fig. 3K). A precursor solution of hydrogel with blue dye was injected into the joint cavity of healthy rats, which were dissected the rats five minutes later. Anatomical photographs showed that the DAGQD@Cu@KGN-SO3−/DA-HA hydrogel and Cu2+-SO3−-HA hydrogel spread across the cartilage surface. After cartilage with DAGQD@Cu@KGN-SO3−-/DA-HA hydrogel was immersed in PBS for 5 min, the hydrogel’s blue color and original appearance remained unchanged. In contrast, the blue color faded, and the outline of the hydrogel disappeared for cartilage with Cu2+-SO3−-HA hydrogel under the same conditions. This indicates that DAGQD@Cu@KGN-SO3−-/DA-HA hydrogel adheres tightly to the cartilage surface, which can be attributed to the abundant catechol structures provided by DA-HA and DAGQD@Cu@KGN SAN. The interface between the DAGQD@Cu@KGN-SO3−-/DA-HA hydrogel and the articular cartilage surface was further observed by a microscope (Fig. 3L, M). The results indicated that there was no gap between the hydrogel and the cartilage surface, demonstrating that the hydrogel adhered well to the cartilage.
The lubrication and adhesion mechanisms of the hydrogels can be attributed to specific factors. The inclusion of SO3−-HA in the DAGQD@Cu@KGN-SO3−/DA-HA hydrogel mimics the natural brush-like lubricating composite nanofibers. SO3− inherently reduces friction by featuring a sulfonate group (akin to lubricin), naturally binding water to hydrate surfaces6. The hydrogel bioadhesiveness stems from DA, drawing inspiration from mussel adhesion (Fig. 3N). The mussels exhibit strong adhesion to diverse substrates in seawater through their secreted mussel foot proteins (Mfps), which are rich in catechol groups that effectively bind to the substrate surfaces28,29. However, catechol groups are prone to oxidation into quinone groups, resulting in the loss of adhesion. To overcome this problem, an antioxidant protein containing cysteine thiol is secreted to reduce the quinone back to the catechol, facilitating long-term adhesion. In essence, the redox reaction between catechol and quinone is an electron transfer mechanism. In this study, DAGQD@Cu@KGN-SO3−/DA-HA hydrogel mimicked the complex adhesion mechanism of mussels. Electrons were transferred from the Cu SAN to the quinone group in DAGQD@Cu SAN, preserving the redox balance between quinone and catechol groups within the hydrogel network. Consequently, the hydrogel retains sufficient catechol groups that interact with the tissue through hydrogen bonds, cation-π interactions, and covalent links, ensuring lasting adhesion.
The mechanical properties of the hydrogels
The mechanical properties of the hydrogel were enhanced by the nanoenhancement effect of the DAGQD@Cu@KGN SAN. The compressive strength (Fig. 3O, P), and anti-swelling properties (Supplementary Fig. 16) of the hydrogel increased with raised SAN content from 0.2 to 1.0 mg mL−1. The increase indicated that elevated SAN content can endow hydrogel with excellent mechanical properties and improved anti-swelling rate by augmenting crosslinking density. However, hydrogel compressive strength, and anti-swelling properties decreased when the SAN concentration was further increased to 2.0 mg mL−1. This declined may be attributed to excess SAN agglomerated within the hydrogel. Thus, hydrogels with optimal compressive performance and anti-swelling properties were obtained at a SAN concentration of 1.0 mg mL−1. SEM images and EDS analysis confirmed that morphology of SAN-SO3−/DA-HA hydrogel consisted of a porous network structure, with well-dispersed SAN and absence of agglomeration (Supplementary Fig. 17). The degradation rate of DAGQD@Cu@KGN-SO3−/DA-HA hydrogel after subcutaneous storage for 28 days was only 7.3%, indicating that the hydrogel had robust stability in vivo (Supplementary Fig. 18). Moreover, sustained release of KGN from the hydrogel network was observed (Supplementary Figs. 19, 20).
In vitro CAT-like activity
DAGQD@Cu-SO3−/DA-HA hydrogel exhibited high CAT-like activity. The CAT-like activity of hydrogels was demonstrated by measuring intracellular oxygen using tris (4,7-diphenyl-1,10-phenanthroline) ruthenium (II) chloride complex ([Ru(dpp)3]Cl2, RDPP). The results demonstrated a decrease in fluorescence intensity following hydrogel treatment (Fig. 4A, B). The fluorescence intensity, ranked from highest to lowest, was as follows: Cu2+-SO3−-HA hydrogel (72.8%), Cu2+-SO3−/DA-HA hydrogel (69.8%), and DAGQD@Cu-SO3−/DA-HA hydrogel (24.2%). The DAGQD@Cu-SO3−/DA-HA hydrogel showed superior CAT-like activity compared with the Cu2+-SO3−/DA-HA hydrogel, due to the efficient CAT-like activity of the DAGQD@Cu SAN. Relative to H2O2 group (85.1%), the fluorescence intensity of the Cu2+-SO3−-HA hydrogel and Cu2+-SO3−/DA-HA hydrogel groups was slightly reduced, which may be attributed to the intrinsic CAT-like activity of Cu2+. Additionally, no significant difference in CAT-like activity was observed between the DAGQD@Cu-SO3−/DA-HA (24.2%) and DAGQD@Cu@KGN-SO3−/DA-HA (24.5%) hydrogel groups, indicating that the KGN had minimal impact on the CAT-like ability of DAGQD@Cu SAN.
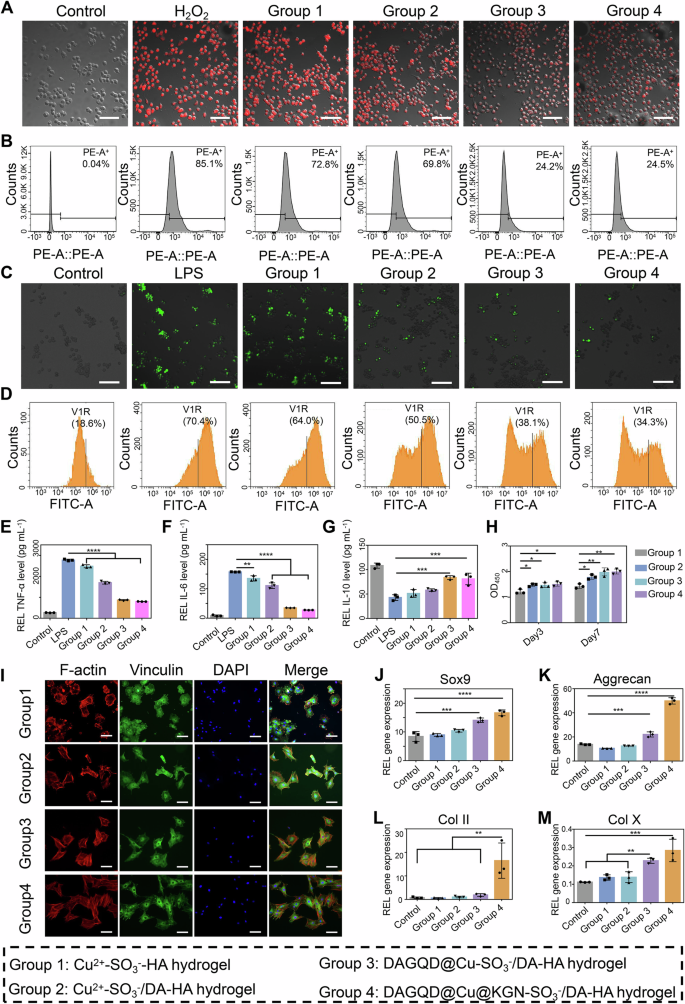
A Intracellular oxygen level of RAW264.7 cells subjected to different treatments was tested using RDPP (n = 3 independent experiments). Scale bar = 100 μm (B) Flow cytometry analysis of RDPP-labeled cells for different groups. C Intracellular ROS staining images of RAW264.7 cells subjected to different treatments (n = 3 independent experiments). Scale bar = 100 μm. D Flow cytometry analysis of ROS-labeled cells for different groups. E–G ELIZA results showed extracellular expression of (E) TNF-α, (F) IL−1β, and (G) IL−10 (n = 3 samples). H BMSCs proliferation on various hydrogels was evaluated using CCK8 after 3 or 7 days of cultivation (n = 3 samples). I Spreading morphology of BMSCs on hydrogel after being cultured for 12 h (n = 3 independent experiments). Scale bar = 200 μm. J–M The expression of cartilage-related markers Sox9, aggregatan (Acan), type II collagen (Col II), and collagen type X (Col X) (n = 3 samples). (Data are presented as the mean ± SD. Statistical analysis was performed using one-way ANOVA followed by Tukey’s post-hoc test, with a value of *P < 0.05, **P < 0.01, ***P < 0.005, and ****P < 0.001).
In vitro ROS scavenging activity
The DAGQD@Cu@KGN SAN endowed the hydrogel with a high ROS scavenging capacity, not only due to the abundance of catechol groups on the hydrogel but also because of the single-atom Cu, which exhibited multiple ROS scavenging and enzyme catalytic activities. Lipopolysaccharide (LPS)-induced macrophages (LPS group) displayed the highest intense fluorescence (70.46%) (Fig. 4C, D). Following hydrogel treatment, the fluorescence intensity decreased. The fluorescence intensity ranked from highest to lowest was Cu2+-SO3−-HA hydrogel (64.07%), Cu2+-SO3−/DA-HA hydrogel (50.54%), and DAGQD@Cu-SO3−/DA-HA hydrogel (38.18%). The slight ROS scavenging capacity of the Cu2+-SO3−-HA hydrogel stemmed from the exposure of a small number of sulfhydryl groups on hydrogel surface. The Cu2+-SO3−/DA-HA hydrogel exhibited better ROS clearance ability than the Cu2+-SO3−-HA hydrogel due to the abundance of catechol groups within it. The DAGQD@Cu-SO3−/DA-HA hydrogel showed an excellent ROS-scavenging effect compared with the Cu2+-SO3−/DA-HA hydrogel, attributed to the efficient SOD and •OH scavenging activities of the DAGQD@Cu SAN. Additionally, nearly no difference was observed in ROS clearance between the DAGQD@Cu-SO3−/DA-HA (38.18%) and DAGQD@Cu@KGN-SO3−/DA-HA (34.30%) hydrogel groups. This indicates that the KGN had minimal impact on the ROS scavenging ability of DAGQD@Cu SAN. In addition, The DAGQD@Cu@KGN-SO3−/DA-HA hydrogel has enhanced cell uptake efficiency attributed to the catechol groups on the surfaces of SAN (Supplementary Fig. 21) and it is non-cytotoxicity even with an increase in SAN concentration from 0.4 to 2.0 mg mL−1 (Supplementary Fig. 22).
In vitro anti-inflammatory activity
The DAGQD@Cu-SO3−/DA-HA hydrogel exhibited excellent anti-inflammatory effects by suppressing M1 macrophage polarization and promoting M2 macrophage activation. The relative expression level of the M1 macrophage-related gene tumor necrosis factor-alpha (TNF-α) and interleukin-6 (IL-6) was evaluated (Fig. 4E, F). The strength of inhibition of TNF-α and IL-6 ranked from high to low was DAGQD@Cu-SO3−/DA-HA hydrogel, Cu2+-SO3−/DA-HA hydrogel, and Cu2+-SO3−-HA hydrogel. The DAGQD@Cu-SO3−/DA-HA hydrogel displayed the highest anti-inflammatory activity, attributed to the collaborative ROS clearance capabilities of the DAGQD@Cu, DA-HA, and SO3−-HA networks. Additionally, the relative expression level of the M2 macrophage-related gene interleukin-10 (IL-10) was evaluated. The results indicated that the DAGQD@Cu-SO3−/DA-HA hydrogel group significantly upregulated IL-10 expression (Fig. 4G), suggesting that the DAGQD@Cu-SO3−/DA-HA hydrogel exerted excellent anti-inflammatory effects by suppressing M1 macrophage polarization and promoting M2 macrophage activation.
In vitro cell proliferation, adhesion and differentiation
The DAGQD@Cu-SO3−/DA-HA hydrogel exhibited favorable properties for BMSCs proliferation, adhesion, and differentiation. Groups 2 (Cu2+-SO3−/DA-HA hydrogel), 3 (DAGQD@Cu-SO3−/DA-HA hydrogel), and 4 (DAGQD@Cu@KGN-SO3−/DA-HA hydrogel groups) demonstrated exceptional proliferation after 3 days of incubation, surpassing that of Group 1 (Cu2+-SO3−-HA hydrogel) (Fig. 4H). This enhanced proliferation was attributed to the excellent cell affinity of catechol in the DA-HA network. After 7 days, the cell numbers in Groups 3 and 4 were significantly higher than those in Group 1, potentially due to the proliferation-promoting effects of GQD and Cu in the DAGQD@Cu SAN30,31.
BMSCs on the surface of Group 1 displayed a circular morphology (Fig. 4I). In Group 2, cell tentacles were observed, indicating improved cell affinity with DA. BMSCs exhibited well-spread morphology on the surfaces of Groups 3 and 4, with larger adhesive spots than on the Cu2+-SO3−/DA-HA and Cu2+-SO3−-HA hydrogels. The spread skeleton and large adhesive spots were attributed to the cell affinity and adhesion of the catechol groups on the surfaces of the DA-HA and DAGQD@Cu SAN. These findings highlight the favorable biocompatibility, cell adhesion, and proliferation activities of DAGQD@Cu-SO3−/DA-HA hydrogels. In addition, DAGQD@Cu@KGN-SO3−/DA-HA hydrogel showed efficient recruitment of BMSCs due to the cell recruitment effect of catechol and KGN on DAGQD@Cu@KGN SAN (Supplementary Fig. 23), suggesting that DAGQD@Cu@KGN-SO3−/DA-HA hydrogel can mobilize BMSCs to migrate into the cartilage defect site.
Furthermore, the expression of Sox9, aggrecan (Acan), type II collagen (Col II), and collagen type X (Col X) in Group 4 (DAGQD@Cu@KGN-SO3−/DA-HA hydrogel) was significantly higher than that in the other groups, illustrating the efficiency of KGN in promoting BMSCs differentiation into chondrocytes (Fig. 4J–M).
RNA sequencing analysis
The results of differential gene analysis showed that there were 785 differential genes between Group 2 and Group 1 (Fig. 5A), 1259 differential genes between Group 3 and Group 1 (Fig. 5B), and 365 genes were the same between the two groups of differential genes (Fig. 5C). Further, the differential genes between Group 3 and Group 1 (894 in total) were analyzed to further explore the mechanism of action of DAGQD@Cu-SO3−/DA-HA hydrogel. GO enrichment analysis found that these genes were enriched in oxidative stress response, autophagy, tissue repair, etc. (Fig. 5D), indicating that the effect of DAGQD@Cu-SO3−/DA-HA hydrogel was significantly correlated with these aspects. GSEA analysis results showed that DAGQD@Cu-SO3−/DA-HA hydrogel can promote the FOXO pathway, inhibit lysosomes, osteoclast differentiation, and TNF pathways, among which the FOXO pathway is related to anti-oxidation, while lysosomes are related to autophagy, osteoclast differentiation is related to cartilage damage, and TNF pathway is related to inflammation (Fig. 5E). Genes related to anti-oxidation, autophagy, repair, and inflammation were selected for further analysis. It can be seen intuitively from the heat map that DAGQD@Cu-SO3−/DA-HA hydrogel has a good effect in promoting the expression of antioxidant-related genes (Sod1, Sod2, Cat, Foxo1, Foxo3, Foxo4), autophagy-related genes (Atg2, Atg3, Atg12), and repair-promoting genes (IL-4 and IL-10). At the same time, it inhibits the expression of pro-inflammatory factors (IL-1a, IL-1b, IL-6) (Fig. 5F). Therefore, DAGQD@Cu-SO3−/DA-HA hydrogel likely reduces the level of cellular oxidative stress and inhibits inflammation through antioxidant effects, while promoting tissue regeneration and removing harmful factors through upregulation of autophagy, ultimately aiding the repair of cartilage defects (Fig. 5G).
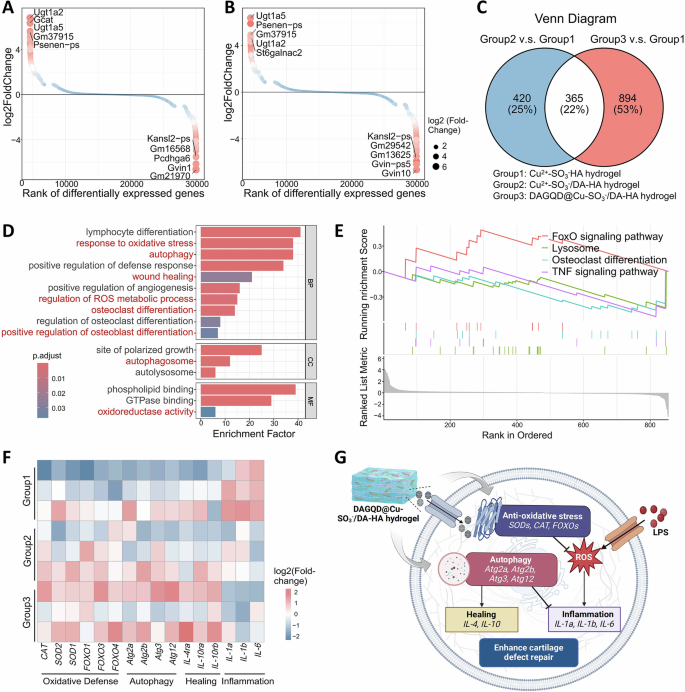
A The different changes of the expression of genes (Group 2 v.s. Group 1). B The different changes of the expression of genes (Group 3 v.s. Group 1) (C) Venn diagram of differentially expressed genes among the three groups. D GO enrichment of sole differentially expressed genes in Group 3. BP, biological processes. CC, cellular component. MF, molecular functions. E GSEA analysis of Foxo signaling pathway, Lysosome, osteoclast differentiation, TNF signaling pathway of DAGQD@Cu-SO3−/DA-HA hydrogel. F Heatmap analysis of differentially expressed genes involved in anti-oxidative stress, autophagy, repairment, inflammation. G Illustrating the potential mechanism by which DAGQD@Cu-SO3−/DA-HA hydrogel enhances cartilage repair and suppresses inflammation.
Hydrogel prevents cartilage wear and tear in RA rats
The cartilage was well protected by the bioadhesive and lubricating DAGQD@Cu@KGN-SO3−/DA-HA hydrogel in early RA within the CIA rat model. The CIA rat model was established through secondary immunization (Fig. 6A). Micro-CT 3D reconstruction results indicated an evident inflammatory reaction in the knee joint of the PBS group, coupled with subchondral bone destruction and hyperostosis (Fig. 6B, Supplementary Fig. 24). Joint injury was partially inhibited by SO3−/DA-HA solution treatment (Group 1), through significant subchondral bone damage persisted. The Cu2+-SO3−/DA-HA hydrogel group (Group 2) exhibited reduced subchondral bone damage compared with the PBS and SO3−/DA-HA solution groups (Group 1), suggesting the ability of the hydrogel to resist cartilage friction to some extent due to its long-term adhesion and lubrication. The destruction of the subchondral bone on the articular surface was effectively suppressed in the DAGQD@Cu-SO3−/DA-HA hydrogel group (Group 3). This outcome stems from the fact that the DAGQD@Cu-SO3−/DA-HA hydrogel not only exhibited local, long-term bioadhesion and lubrication but also exhibited exceptional antioxidant and anti-inflammatory effects for RA treatment. Importantly, no signs of cartilage collapse or damaged boundaries were observed in the DAGQD@Cu@KGN-SO3−/DA-HA hydrogel group (Group 4). The joint surface of rats in Group 4 closely resembled that of healthy rats, illustrating the excellent bioadhesion, lubrication, and cartilage repair capabilities of the DAGQD@Cu@KGN-SO3−/DA-HA hydrogel. Moreover, the DAGQD@Cu@KGN-SO3−/DA-HA hydrogel does not affect the joint movement (Supplementary Fig. 25).
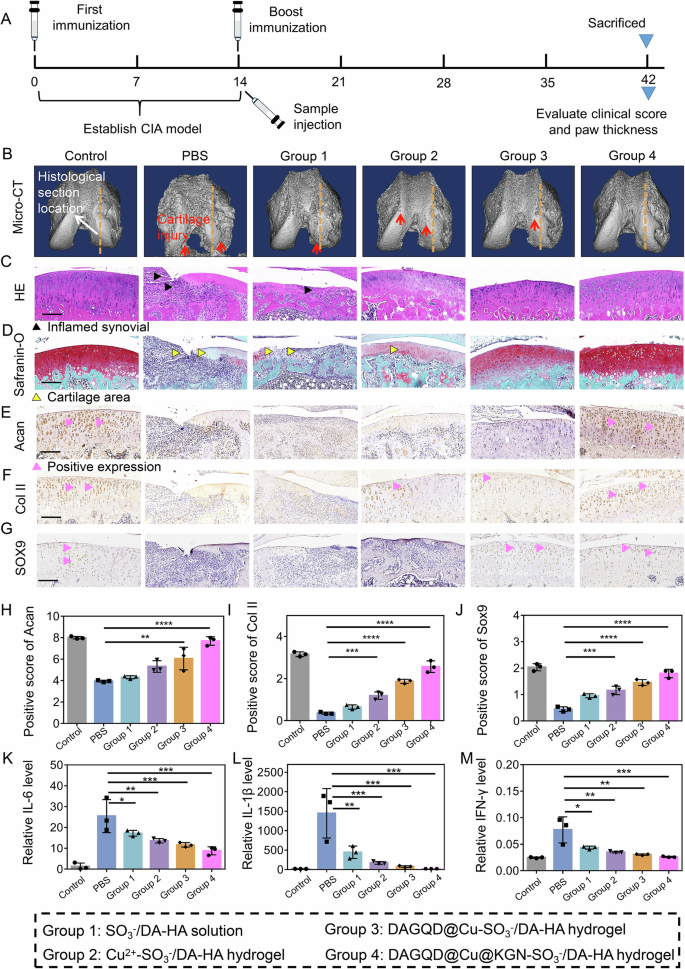
A Schematic illustration of the experimental procedure. B Micro-CT images of rat knee joints after 4 weeks of treatment. The yellow dotted line represents the location of the histological section of the rat. C, H, E and (D) Safranine-O stain images of rat knee joints after 4 weeks of treatment (n = 3 independent experiments). E–G Immunohistochemical staining of cartilage markers (Sox9、Col II and Acan). The black symbol represents the positive expression. Scale bar = 200 μm. H–J The quantitative analysis of cartilage markers (Sox9、Col II and Acan) (n = 3 samples). K–M Levels of IL-6, IL−1β, and IFN- γ in rat serum after 4 weeks of treatment (n = 3 samples). (Data are presented as the mean ± SD. Statistical analysis was performed using one-way ANOVA followed by Tukey’s post-hoc test, with a value of *P < 0.05, **P < 0.01, and ***P < 0.005).
Inflammation was effectively alleviated by bioadhesive and lubricating hydrogels in RA. The results showed that the SO3−/DA-HA solution (Group 1), Cu2+-SO3−/DA-HA hydrogel (Group 2), DAGQD@Cu-SO3−/DA-HA hydrogel (Group 3), and DAGQD@Cu@KGN-SO3−/DA-HA hydrogel (Group 4) all exhibited an RA-suppressing effect, accompanied by the reduction in swelling of hind paws, as compared with PBS-treated CIA rats (PBS group) (Supplementary Fig. 26A, B). The clinical arthritis scores were consistent with the severity of arthritis in the paws (Supplementary Fig. 26C). The DAGQD@Cu@KGN-SO3−/DA-HA hydrogel group (Group 4) demonstrated the highest efficacy in suppressing paw swelling and clinical arthritis scores, indicating that the DAGQD@Cu@KGN-SO3−/DA-HA hydrogel effectively alleviated inflammation in RA.
In addition to its anti-inflammatory activity, the hydrogel exhibited excellent cartilage repair ability. The H&E staining results showed that the PBS-treated CIA rat joints had a large amount of inflammatory cell infiltration accompanied by severe erosion and thinning of the cartilage layer (Fig. 6C). Safranin O staining of PBS-treated CIA rat joints showed cartilage destruction and stratification, with a significant decrease in cartilage layer thickness (Fig. 6D). These results indicated that the joint cavity of CIA rats can form an inflammatory storm in the short term, leading to significant degradation of the joint cartilage. Groups 3 (DAGQD@Cu-SO3−/DA-HA hydrogel) and 4 (DAGQD@Cu@KGN-SO3−/DA-HA hydrogel groups) elicited excellent effects against RA-induced inflammatory infiltration compared with Groups 2 (Cu2+-SO3−/DA-HA hydrogel) and 1 (SO3−/DA-HA solution group), attributed to the excellent ROS clearance ability of the DAGQD@Cu@KGN SAN. Safranin-O staining images showed that the protein polysaccharides in the cartilage matrix of Group 4 were significantly increased and were higher than those in Groups 3, 2, and 1 with fewer protein polysaccharides. Moreover, the cartilage thickness and structure in Group 4 were closer to those of normal rats (control group).
The immunohistochemical staining analysis revealed a significant reduction in the expression of cartilage proliferation and differentiation-related proteins Sox9, Col II, and Acan in the PBS group compared with normal rats. This observation suggests that PBS-treated CIA rats exhibited significant cartilage wear characteristics (Fig. 6E–J). The expression levels of Sox9, Col II, and Acan in the SO3−/DA-HA solution group (Group 1) were lower than those in the Cu2+-SO3−/DA-HA hydrogel group (Group 2), indicating that the long-term bioadhesion and lubrication properties of the hydrogel play a crucial role in cartilage protection. The expression levels of Sox9, Col II, and Acan were higher in the DAGQD@Cu-SO3−/DA-HA hydrogel group (Group 3) than in the Cu2+-SO3−/DA-HA hydrogel group (Group 2). This difference can be attributed to the excellent antioxidant activity of DAGQD@Cu SAN, effectively alleviating inflammation in RA and mitigating cartilage damage. Remarkably, the DAGQD@Cu@KGN-SO3−/DA-HA hydrogel group (Group 4) showed the highest expression levels of Sox9, Col II, and Acan. This enhancement is attributed to the promotion of cartilage differentiation by KGN in the SAN.
The cartilage repair ability of the hydrogel was attributed to its immunoregulatory activity (Supplementary Fig. 27). The expression of M1 related inflammatory factors (TNF-α and IL-6) was highest in PBS-treated CIA rat joints. The expression of TNF-α and IL-6 were slightly inhibited after treatment with SO3−/DA-HA solution (Group 1) and Cu2+-SO3−/DA-HA hydrogel (Group 2). This is due to the antioxidant capacity of the catechol structure on the surface of the DA-HA network. Moreover, the expression of TNF-α and IL-6 was significantly reduced in SAN-contained groups (DAGQD@Cu-SO3−/DA-HA hydrogel and DAGQD@Cu@KGN-SO3−/DA-HA hydrogel), whereas the expression of M2 related anti-inflammatory factor IL-10 was significantly increased, illustrating that SAN has excellent ROS scavenging ability, and can regulate the RA-induced inflammatory microenvironment by promoting M1 to M2 transformation. Moreover, the DAGQD@Cu@KGN-SO3−/DA-HA hydrogel not only inhibited systemic inflammation in CIA rats (Fig. 6K–M), but also did not cause systemic toxicity (Supplementary Fig. 28, 29).
Hydrogel promotes cartilage reconstruction in RA rabbit
The DAGQD@Cu@KGN-SO3−/DA-HA hydrogel demonstrates a significant capacity for reconstructing severe cartilage defects in rabbits with RA. First, a complex model, combining cartilage and subchondral bone defects with RA, was established in rabbits (Fig. 7A). After 8 weeks of induction, the osteochondral defect in the PBS group was severely corroded, with inflammation spreading to the surroundings and bottom of the defect (Fig. 7B). Following treatment, inflammation was alleviated, and the defect underwent repair. Micro-CT scanning (Fig. 7C) and histopathological (Fig. 7D, E) analyzes confirmed the expansion of the defect area and inflammation surrounding the defect in the PBS group. In contrast, the DAGQD@Cu@KGN-SO3−/DA-HA hydrogel treatment demonstrated the most efficient defect repair and inflammation inhibition, followed by the DAGQD@Cu-SO3−/DA-HA hydrogel, Cu2+-SO3−/DA-HA hydrogel, and SO3−/DA-HA solution. The defect showed partial repair, and inflammation was slightly alleviated after the SO3−/DA-HA solution treatment (Group 1). This can be attributed to the antioxidant capacity of catechol in the DA-HA network.
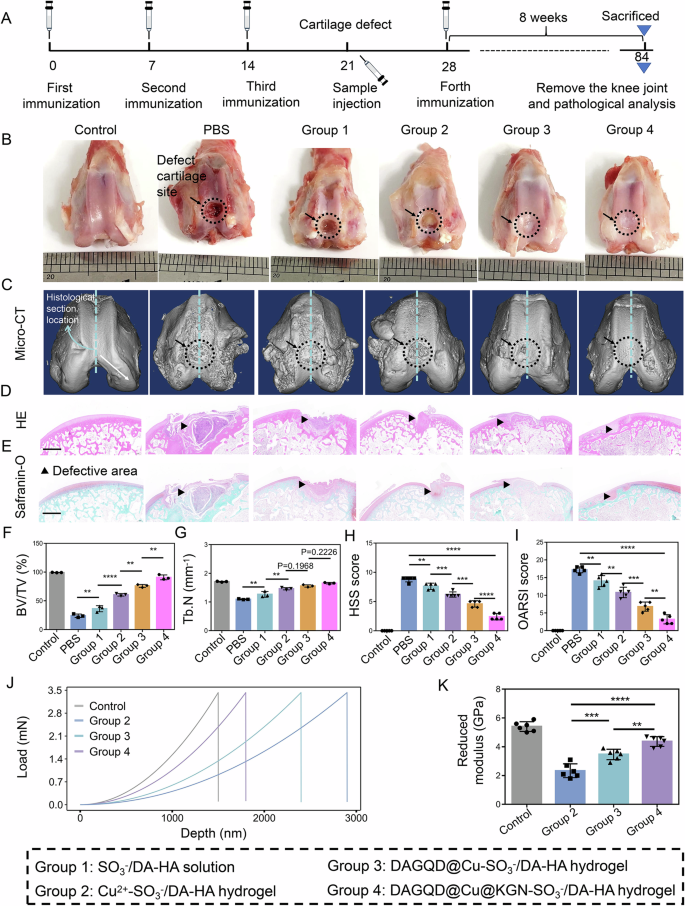
A Schematic illustration of the experimental procedure. B Macroscopic observation of repaired cartilages at 8 weeks (black arrow: defect cartilage site). C Micro-CT images of rabbit’s knee joints after 8 weeks of treatment. The blue dotted line represents the location of the histological section of the rabbit. D, H, E Safranine-O stain images of the rat knee joints after 8 weeks of treatment (n = 3 independent experiments). Scale bar = 2 mm. F Quantitative analysis of bone volume over total volume ratio (BV/TV) and (G) trabecular number (Tb.N) of CIA rats in different treated groups (n = 3 samples). H Histological synovitis scoring (HSS) and (I) modified Osteoarthritis Research Society International (OARSI) score of rabbit knee joints after 8 weeks of treatment (n = 5 samples). J Indentation test results and (K) corresponding reduced modulus of the newly formed tissues in different groups (n = 6 samples). (Data are presented as the mean ± SD. Ordinal data such as HSS score and OARSI score was analyzed by Kruskal-Wallis test and continuous data was analyzed by one-way ANOVA followed by Tukey’s post-hoc test, with a value of **P < 0.01, ***P < 0.005, and ****P < 0.001).
After the hydrogel treatment, the defect diameter and depth decreased, and the degree decreased from high to low in Groups 4 (DAGQD@Cu@KGN-SO3−/DA-HA), 3 (DAGQD@Cu-SO3−/DA-HA), and 2 (Cu2+-SO3−/DA-HA hydrogels). The slight recovery of the cartilage and subchondral bone in Group 2 was attributed to the ability of Cu to promote cartilage differentiation32. Group 3 showed better cartilage repair ability than the Group 2 because of the exceptional antioxidant capacity of DAGQD@Cu SAN, which promoted self-repair of the matrix by regulating the inflammatory microenvironment. Group 4 displayed the best cartilage repair ability. This is because the released KGN promotes cartilage reconstruction by recruiting endogenous BMSCs, except for the excellent anti-inflammatory activity of DAGQD@Cu SAN in the DAGQD@Cu-SO3−/DA-HA hydrogel. Quantitative analysis of the micro-CT images also revealed progressive improvements in both the bone volume to total volume ratio (BV/TV) at the defect site (Fig. 7F) and the trabecular number (Tb.N) (Fig. 7G) within the DAGQD@Cu@KGN-SO3−/DA-HA hydrogel group (Group 4). Moreover, the histological synovitis scoring (HSS) score and Osteoarthritis Research Society International (OARSI) score exhibited a significant decrease following treatment with the DAGQD@Cu@KGN-SO3−/DA-HA hydrogel in Group 4 (Fig. 7H, I). The mechanical test results of the newly formed organization showed that the deformation resistance and reduced modulus of the newly formed tissues in the DAGQD@Cu@KGN-SO3−/DA-HA hydrogel group (Group 4) were significantly higher than those in the other treatment groups and close to those in the control group (normal rabbit without hydrogel treatment) (Fig. 7J, K). DAGQD@Cu@KGN-SO3−/DA-HA hydrogel group (Group 4) exhibited superior cartilage repair ability, which can be attributed to the KGN-mediated promotion of cartilage reconstruction, coupled with the excellent anti-inflammatory activity of DAGQD@Cu SAN in the hydrogel.
The cartilage reconstruction mechanism of the DAGQD@Cu@KGN-SO3−/DA-HA hydrogel in rabbits with RA can be summarized as follows: (1) The abundant catechol groups in DA provided the hydrogel with long-term adhesion to the cartilage surface. (2) The SO3− endowed the hydrogel with lubricity, preventing further cartilage wear and tear in RA. (3) The GAGQD@Cu SAN alleviated the inflammatory microenvironment in RA joint cavity due to its excellent ROS-scavenging activity. Moreover, Cu2+ in the SAN demonstrated a certain ability to promote cartilage differentiation. (4) KGN triggered the differentiation of BMSCs into chondrocytes by recruiting endogenous host BMSCs guiding them to mitigate and target the damaged cartilage affected by RA, thus contributing to its repair.